 |
 |
 |
|
 |
|
Succesfull
Photometric Observation of Exoplanet HD 209458b
at CBA Belgium Observatory
|
This
is shortened and slightly reworked version of
an article on exoplanet astronomy that will
appear in a future edition of "Heelal",
a monthly publication of the Belgian
Astronomical Association VVS. The article is
called "Sterbedekkingen door
exoplaneten" (authors : Siegfried
Vanaverbeke and Tonny Vanmunster).
|
|
1.
Introduction
The American
astronomer Otto Struve was the first person
suggesting (in 1952) the possibility of observing
exoplanets through stellar occultations. In a short
article in The Observatory [1] he concluded
that it should be possible to indirectly detect
massive short-period planets by observing their
transits : 'We know that stellar companions can
exist at very small distances. It is not
unreasonable that a planet might exist at a distance
of 1/50 A.U., or about 3,000,000 km. Its period
would be about 1 day. There would of course also be
eclipses. Assuming that the mean density of the
planet is five times that of the star (which may be
optimistic for such a large planet) the projected
eclipse area is about 1/50th of that of the star,
and the loss of light in stellar magnitudes is about
0.02. This, too, should be ascertainable by modern
photoelectric methods, though the spectrographic
test would probably be more accurate. The advantage
of the photometric procedure would be its fainter
limiting magnitude compared to that of the
high-dispersion spectrographic technique.'
Until 1995,
exoplanets remained hypothetical objects. That year,
however, astronomers discovered a first exoplanet,
orbiting 51 Pegasi, based on Doppler measurements
[2].
In November 1999, two
independent teams of astronomers succeeded in
discovering the first transit of an exoplanet, through photometric observations of HD 209458,
a star of magnitude 8 in Pegasus. One of the teams
was headed by Prof. Tim Brown of Harvard University.
His PhD student David Charbonneau obtained transit observations using a telescope with an
aperture of 10-cm only. Evidently, this opened
perspectives for amateurs to enter the domain of
exoplanet transit photometry. |
|
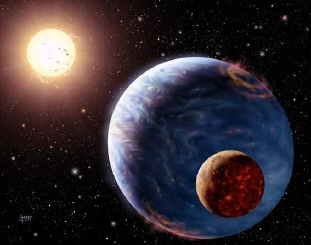
|
|
|
Drawing
by David
A.Hardy of a planetary system
orbiting Tau Bootis. The exoplanet has
approximately 4x the mass of Jupiter. |
2. Transit
observation of HD 209458 at CBA Belgium Observatory
The Finish amateur
astronomer Arto
Oksanen, member of CBA Finland, was the first
amateur to
observe a photometric transit of HD 209458b, at his Nyrola Observatory in
September 2000. In general, observers with small telescopes, a CCD
camera and a high-quality photometric procedure are
able to make exoplanet transit observations,
provided they can reach a photometric precision of
minimal 1% (see further). To foster pro-am
collaborations on exoplanet research, Transitsearch.org
has been created a few years ago (see further). The
goal of Transitsearch.org is to coordinate and
direct a cooperative observational effort which will
allow experienced amateur astronomers and small
college observatories to discover transiting
extrasolar planets.
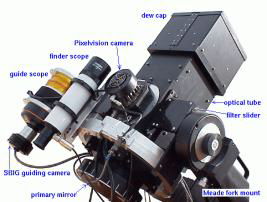 |
|
The exoplanet of HD
209458 is creating an eclipse every 3.52 d. At first
sight, it therefore seems there are plenty of
opportunities to observe such an event. However, if
we take into account
that preferably observations cover a complete transit from
ingress till egress, and that the altitude of the
star has to be 30 degrees or more (a lower altitude
will result in too much noise being introduced in
the photometric lightcurve), one immediately
realises that chances are rather limited. Add to
this the poor weather conditions of Belgium in
general, and in practice only a few occasions per
year remain. |
Eclipses
of HD 209458 were discovered using the STARE
telescope (STellar Astrophysics and Research
on Extrasolar planets). |
|
|
Successful
observation on 2003, September 3rd
I made a first
attempt to observe a transit of HD 209458b on August
27th, 2003, using the 0.35-m f/6.3 telescope at CBA
Belgium Observatory. The CCD camera was an SBIG
ST-7, without filters. I made differential
photometric observations, comparing the magnitude of
HD 209458 with one or more constant comparison
stars. The attempt was unsuccessful,
mainly because I did not have access to a good
comparison star on the CCD images (see
further). The
night of September 3rd, 2003 was clear again, and
allowed me to successfully measure a complete
transit. Armed with my experiences of the failed
trial on August 27th, I started the observing
session on 22h42m UT (Sep 3.946 UT), i.e. 1 hour
prior to the predicted time of ingress. After 4h42m
and 1022 CCD images, the session was concluded. At
that moment, HD 209458 was less than 30 degrees
above the local horizon. Analysis
of the observations Over
the years, I have built up quite some experience in
analysing photometric observations of cataclysmic
variables, by participating in many of the CBA
campaigns. My first approach therefore was to apply
these same techniques to the analysis of my HD
209458b observations. The day after my observing
session, I made a first attempt, using AIP4WIN to
perform aperture photometry (with flat fielding,
bias and dark frame reductions properly applied).
The result was rather discouraging : the light curve
did not show modulations that could point to a
successful transit observation. I reached a
photometric accuracy of 0.01 - 0.02 mag only, and knew from
literature that about 0.005 mag (5 millimagnitudes)
would be required. It
would take several more weeks before I arrived at a
useful analysis method, that allowed to process
all CCD images and to finally deduct the light curve
below.
|
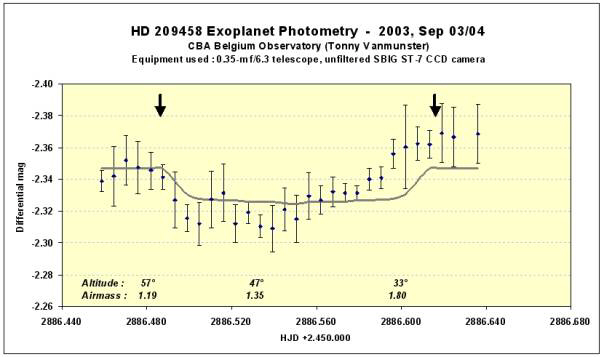 |
|
|
Photometric
observations of exoplanet HD 209458b,
obtained on September 03, 2003 at CBA Belgium
Observatory.
(c) Tonny Vanmunster |
|
A
first roadblock in the data analysis was the spread in accuracy of the
photometric reductions of individual CCD frames. To
achieve a 0.005 mag precision, I had to apply some
form of 'statistic sampling'. This was done
by adding successive raw CCD images to build
a new raw 'master image'. I then
performed aperture photometry on these master
images. I tried several sample-sizes, ranging
from 5 to 72 images. I obtained best results by
adding 30 CCD images each time to create a master
image. Unfortunately, AIP4WIN has no automated way
to simply add CCD images. I therefore decided to
write some software myself, to automate the whole
process. This
first approach almost immediately led to a much
improved light curve, showing the 'signature' of an
exoplanet transit. A
next optimisation was obtained by aligning
the raw CCD images, prior to adding them. This
ensures that stars appear at more or less identical
(pixel) positions before adding images. This
technique compensates for small inaccuracies in the
telescope mount. Unfortunately, AIP4WIN again has no
automated mechanism for this, so I had to use own
(Visual Basic) software and commercial packages to
get the work done. A
final step towards further increasing the accuracy
of my photometric method was to use 'ensemble
photometry'. This techniques aims at grouping a
number of constant comparison stars into 1 'virtual'
comparison star that then is used in the
photometric reduction. This approach averages noise
levels introduced by the comparison stars. AIP4WIN only supports the use
of 1 comparison (and 1 check) star. I bought a copy of
MIRA A/P, which is a more
professional photometry software, that supports
ensemble photometry. Using Mira A/P, I arrived at a slightly
improved light curve. The
final result of my data analysis efforts is shown in the
light curve above. It depicts the ingress, eclipse
and egress of the exoplanet. Each dot in the curve
shows the (differential) magnitude of HD 209458 for
30 (combined) CCD images, as compared to the
comparison star HD 209346. Error bars are included
as well. The grey curve shows the theoretical
transit lightcurve. Predicted times of ingress and
egress are marked with arrows. Note that the
photometric accuracy is clearly declining towards
the end of the observation session, due to the low
altitude of HD 209458.
3.
Be
prepared ...
Interested in
observing an exoplanet transit yourself ? Here are
some further tips to share. We already explained
some improvement actions to increase the photometric
accuracy of your CCD images (e.g., through statistic
sampling). However, those are 'a posteriori'
techniques. Equally or even more important, are
techniques that allow to ensure a good photometric
accuracy during image acquisition.
Focus : we
know from experience that a slight defocusing of CCD
images somewhat improves photometric accuracy, due
to the fact that the light of a star is spread over
a larger CCD area. The impact of course depends on
the focal ratio of your telescope, and on the used
CCD binning method. I always use 2x2 binning.
Comparison stars
: the selection of appropriate comparison stars is a quite fundamental
step (see also our
failed first trial on August 27th, 2003). Select a
comparison star of a similar magnitude and color as
the observed star. This is not always easy to
realise : HD 209458 has V magnitude 7.65, and we
used HD 209346 (V = 8.32) as a suited comparison
star. Color differences are especially important
when doing unfiltered photometry of low-altitude
objects.
Exposure time
: the optimal exposure time for your CCD images needs to be determined
by trial and error. Too short exposures will result in insufficient pixel counts, and
reduce the photometric accuracy below the required level of 1%. Too long exposures
have a risk of saturating pixels, making the photometric reduction useless. In
general, one should aim at an exposure time that results in achieving about 40% to
50% of the so called 'full well capacity' of your CCD camera. For an SBIG ST-7,
this corresponds to a pixel count of approx. 30.000.
Scintillation : evidently, atmospheric turbulences have a quite negative influence
on photometry. Scintillation is most evident when exposure times are below 5 seconds.
With longer exposures (10 sec or more), the effect almost disappears. Scintillation
probably accounts for most of the error range introduced in our HD 209458b light curve
above. Too avoid saturation, by having to use exposure times of more than 10
seconds, one might consider the use of a V-filter.
Instrumental errors : a final source of errors
is due to mechanical or electronic limitations of your setup. This category encompasses for instance small
inaccuracies in a telescope mount, causing CCD images not to perfectly overlap. These
errors can be easily reduced by aligning CCD images before stacking (see above).
Another member of this category of errors are temperature changes over the course of
a night. Especially with SCT telescopes, this may result in a gradual defocusing.
If not compensated for, CCD imaging might become useless after some time.
4. The Transitsearch Project
The study of exoplanets meanwhile has become one of the fastest growing research areas
in contemporary astronomy. Amongst others, this has resulted in the set up of an
international collaboration project to search for, and study exoplanets, called
Transitsearch (see www.transitsearch.org for full details). Transitsearch has been
initiated by Gregory Laughlin (University of California, Santa Cruz) and Tim
Castellano (NASA Ames Research Center). The Transitsearch website has a list of
target stars to monitor and predicted times of transit, including indications on
the uncertainties of the predictions, on the probabilities of a transit, etc.
In addition, the website contains interesting information on observing techniques
(CCD photometry) and a discussion forum.
Good luck with your
transit observations !
Acknowledgements
I wish to thank
Siegfried Vanaverbeke (Belgium) for the many
inspiring email exchanges in preparing for the
exoplanet observations, and during the analysis
afterwards.
References [1] Stryve, O., The Observatory, 72,
199-200 (1952).
[2] Mayor, M., Queloz, D., Nature,
378, 355 (1995).
|
|
|
 |
|
 |
|
|
 |
Copyright © 2004 - Tonny Vanmunster.
|
 |
|
 |